A new type of semiconductor that can store information using electric fields may lead to...
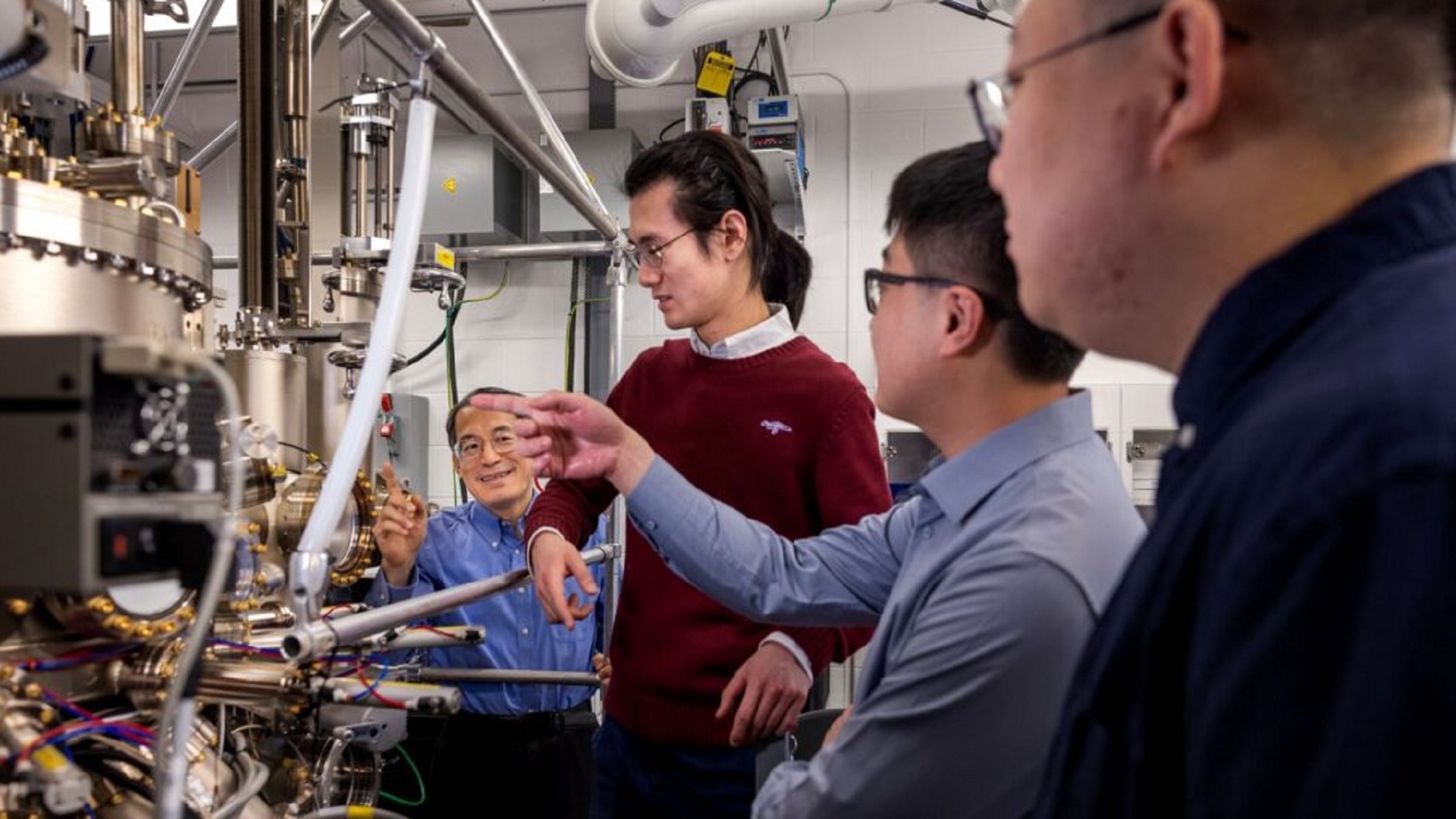
A new type of semiconductor that can store information using electric fields may lead to more energy-efficient computers, ultra-precise sensors, and technologies that convert signals between electrical, optical, and acoustic forms.
However, scientists had long been puzzled by how these materials could sustain two opposing electric polarizations without breaking apart.
A team of engineers at the University of Michigan has now uncovered why the materials, known as wurtzite ferroelectric nitrides, don’t tear themselves apart.
“The wurtzite ferroelectric nitrides were recently discovered and have a broad range of applications in memory electronics, RF (radio frequency) electronics, acousto-electronics, microelectromechanical systems, and quantum photonics, to name just a few. But the underlying mechanism of ferroelectric switching and charge compensation has remained elusive,” said Zetian Mi, the study’s co-corresponding author.
“How is the material stabilized? It was largely unknown.”
How broken bonds hold the structure together
Using advanced electron microscopy and quantum calculations, the researchers found that when opposing electric polarizations collide inside the material, the crystal structure momentarily fractures, creating dangling atomic bonds.
Instead of weakening the structure, these broken bonds provide just the right charge balance to keep the material stable, acting like an atomic-scale glue.
Like magnets, polarized materials have positive and negative ends. In these semiconductors, that polarity can flip under an electric field, and stay that way even after the field is gone.
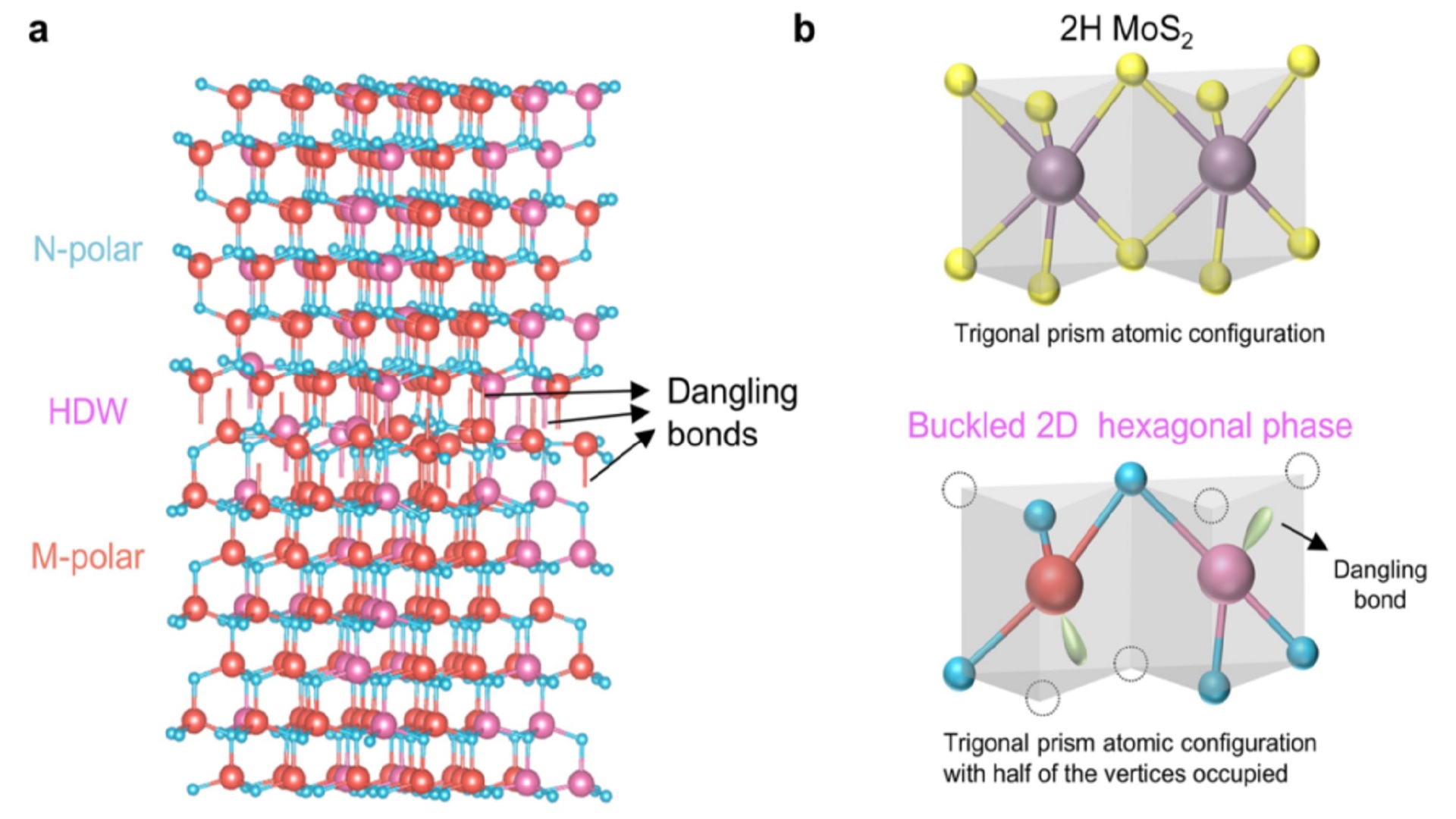
But the material often splits into regions with opposite polarizations, and scientists long puzzled over why it didn’t crack where the like charges met.
Atomic-level bonds enable high-conductivity
“It’s a simple and elegant result—an abrupt polarization change would typically create harmful defects, but in this case, the resulting broken bonds provide precisely the charge needed to stabilize the material,” said Emmanouil Kioupakis, a U-M professor of materials science and engineering and a co-corresponding author of the study.
“What’s remarkable is that this charge cancellation isn’t just a lucky accident—it’s a direct consequence of the geometry of tetrahedra,” he said. “This makes it a universal stabilizing mechanism in all tetrahedral ferroelectrics—a class of materials that’s rapidly gaining attention for its potential in next-generation microelectronic devices.”
Electron microscopy revealed the atomic structure of scandium gallium nitride, showing how the domains’ meeting points caused the usual hexagonal structure to buckle, creating broken bonds. These bonds, closer than normal, form a structure further understood through density functional theory calculations.
A key to high-power, high-frequency electronics
These dangling bonds stabilize the material and act as a conductive channel for electricity, carrying about 100 times more charge than typical gallium nitride transistors. This channel can be controlled by adjusting the electric field, offering a tunable pathway for current flow.
Recognizing its potential as a field-effect transistor, the team aims to develop this material for high-power, high-frequency electronics. The device was crafted at the Lurie Nanofabrication Facility and analyzed at the Michigan Center for Materials Characterization.
The study has been published in the journal Nature.